Scottish hydrogen: assessment report
Examines how applications of hydrogen-based technologies in transport, industry, heat and whole system approaches can best be deployed in Scotland.
4 Hydrogen Techology
4.1 Hydrogen solutions
Hydrogen has broad applications across the energy system with numerous ways to produce, move, store and use hydrogen. These are described in more detail, including their technology maturity, in Appendix A2.2.
The global energy system has always been, and will likely continue to be, multi-vector i.e. energy is produced and supplied in a diversity of ways dominated by molecules and electrons. An over reliance on any one energy vector is likely to reduce the overall system resilience.
Understanding the mix of technologies deployed in a future net-zero energy system is complex and it is likely that a range of solutions will exist which are dependent on location specific applications. A range of factors to consider are summarised in Table 4 and discussed in the context of hydrogen solutions below
“Hydrogen technology is already a well-established vector globally”
Stakeholder questionnaire opinion
Table 03: Factors influencing technology deployment in a net-zero energy system
Technology Maturity/ Readiness And Risk
Hydrogen technologies are at various stages of maturity across different applications. In the hydrogen supply chain, some technology applications are well proven and already used extensively, like SMR without CCUS. Others are still in the demonstration phase, for example fuel cell use in heavy goods vehicles and rail.
User Requirements, Consumer Choice And Consumer Acceptance
Whether consumers accept and want to use hydrogen, will be key to how much it is used in the economy. One key advantage of hydrogen is that, as a gas, it often gives a similar user experience to the hydrocarbons solutions it will displace. For example, hydrogen fuel cell vehicle refuelling is more similar to diesel/petrol vehicles, than electrical vehicles that require much longer charging durations.
Commercial Competitiveness, Cost Base And Whole System Analysis
The commercial competitiveness of hydrogen relative to the hydrocarbons they are displacing and alternative decarbonisation methods will determine the extent of hydrogen’s role in the energy system. Understanding the best decarbonisation solution for an application is complex and requires an understanding of the location specific attributes of those applications, the cost trajectories of energy commodities and carbon, and the context of the impact on the wider system. Hydrogen is likely to confer benefits when considered in a wider system analysis. It provides more overall system flexibility, storage capacity and better utilisation of existing infrastructure.
Investment Case
The investment case for any infrastructure project considers lifecycle cost (CapEx and OpEx), revenue/income, pay back periods and market risk. Currently decarbonisation solutions, including hydrogen, are generally not cost competitive with hydrocarbon alternatives. Until the market matures and cost reductions are realised market support/subsidy is likely to be critical to support hydrogen business models.
Socioeconomic Benefit
A Just Transition is not just about focusing on delivery of the lowest cost decarbonisation solution. It also needs consideration of the creation of jobs and economic benefits associated with the transition. Net-zero will result in a major shift away from a carbon based economy into a new green economy, requiring different skills and economic activities. Early movers in hydrogen are likely to capture more economic benefits by securing these new activities. However, it is important to be realistic and focused on where economic value can be captured, considering indigenous strength relative to global capability and the scale of the domestic industry.
Geography
Geography has a key role to play in how any hydrogen economy will develop, and it is possible that solutions will be region and location specific. The cost of hydrogen at point of demand depends on the local cost base of production, the way in which it is distributed and stored, as well as the cost base of the energy source it is displacing. Rural and island locations often have high fuel costs and local constrained renewable production which provide economically favourable conditions for hydrogen as a solution. Urban centres are generally not co-located next to production, but do have more developed existing infrastructure, and larger volumes of demand to stimulate hydrogen production at scale.
4.2 Hydrogen energy costs
Production Costs
Some hydrogen technologies are mature with established costs, others are relatively novel and therefore offer significant opportunities for cost reduction. As the use of hydrogen increases, economies of scale offer an opportunity to decrease the unit cost and therefore the price of hydrogen delivered to applications.
Currently hydrogen production costs vary significantly based on the production method and production location. Multiple recent international studies have attempted to estimate how both blue and green hydrogen production cost will evolve globally out to 2050. The uncertainty of how these costs will evolve means that estimates can vary substantially.
This is particularly true for green hydrogen production, as it is less mature and reliant on renewable electricity costs, which itself has a wide cost range. Most studies conclude that blue hydrogen will have a lower cost over the next decade or so, with green hydrogen potentially competing with blue from the mid-2030s onwards.
"Hydrogen technology is already a well-established vector globally"
Stakeholder questionnaire opinion
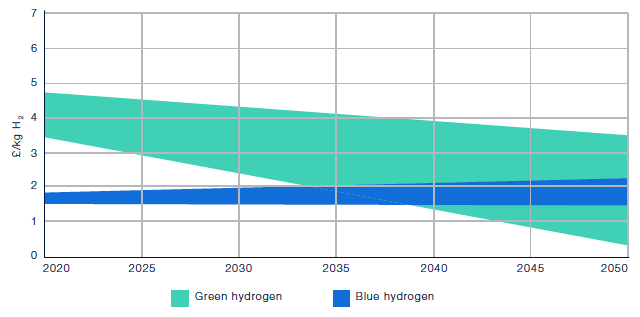
Blue hydrogen costs are essentially the wholesale natural gas price plus a production 'premium'. This covers the efficiency loss (i.e. the additional kilowatt hour (kWh) of natural gas required for each kWh of hydrogen produced) the cost of transporting and storing the carbon and the operational and capital costs of the plant itself. Blue costs are expected to increase out to 2050 as the cost of natural gas rises and potential carbon taxes are introduced.
To limit the need to construct additional natural gas pipelines, blue hydrogen production will need to be near large natural gas sources or import points.
It will also need to be in proximity to CCUS facilities, or at least be connected to any future CCUS networks.
Green hydrogen costs are also mostly determined by the feedstock costs, in this case renewable electricity. Costs also include the capital expenditure CapEx and operational expenditure OpEx and efficiency of the electrolyser system.
The reducing cost of renewable electricity, increased scale of electrolyser manufacture and improved efficiencies mean that the cost of green hydrogen is expected to reduce substantially out to 2050.
Figure 16 shows impacts of electricity price on hydrogen production costs in 2035 shows how significantly green hydrogen production is impacted by electricity price. Using today's electricity price with a forecast CapEx and OpEx for 2035 results in a hydrogen cost of over £3/kilogram (kg), with the feedstock electricity accounting for over 70% of the total hydrogen cost. This is compared to a hydrogen cost of under £2/kg if the electricity can be sourced at 2p/kWh. For Scotland, driving down the cost of offshore and onshore wind electricity production, will be key to cost effective green hydrogen production.

Hydrogen transportation and storage costs
Transmission and distribution
Production costs are only part of the story, the costs of transporting the hydrogen from production to end usage application is important for determining the overall costs. Currently, most hydrogen production is co-located with end use. In the future this will not be practical for all end uses.
It is expected that transmission and distribution pipelines will be the lowest cost option in moving hydrogen from production source to end user. The capital costs of building new transmission pipelines or a new distribution network is a significant investment and would require substantial volumes for it to be viable. However, if the existing network could be converted then much of these costs could be avoided.

The potential to use the existing gas network is one of the main attractions for using hydrogen in Scotland and the UK, which has a relatively well-developed gas network compared to many other countries.
Transporting gas overland by rail or lorry results in significantly higher operational cost than pipelines, but offers much more flexibility supplying smaller users where the capital expense of pipelines would not be justified[36, 37]. All transportation technologies are described in more detail in Appendix A2.2.
Storage
As a gas, hydrogen offers significant advantages over electricity when it comes to storage, particularly long term seasonal storage between summer and winter. Figure 18 provides a summary of the different hydrogen storage options.
Storing hydrogen does pose additional challenges compared to natural gas due to its relatively low energy density. It is estimated that if hydrogen were to completely replace natural gas in the world economy today, total storage infrastructure would need to increase 3-4 times[13].
Compressed gas hydrogen storage is more expensive than storing hydrogen in a salt cavern or other underground facilities on a per kilogram basis. However, the very high upfront cost of underground storage means that it is only available once hydrogen production has reached sufficient scale to warrant such an investment. Liquified hydrogen storage has higher capital and operational costs than compressed hydrogen but it is used when space is a key concern e.g. to transport hydrogen.
All storage technologies are described in more detail in Appendix A2.2.

Conversion to ammonia
Ammonia offers a potential option for both transporting and storing hydrogen. Ammonia has a much higher energy density than gaseous or liquid hydrogen, which may make it an attractive mechanism for both transporting and storing hydrogen. There is already a large worldwide market for ammonia, and it is regularly transported in large quantities[37]. However, this needs to be balanced against the additional cost, energy requirements and any associated emissions from the conversion of hydrogen to ammonia, and reconversion back to hydrogen at the point of use[37].
4.3 Hydrogen supply and demand balance
Scotland's energy demand
Scotland's current energy demand equates to approximately 160 TWh[38], circa 10% of the UK's total energy demand of 1,640 TWh[39]. The Scottish Energy Strategy[40] estimates that total energy demand by 2050 will decrease by 5-28%, to 110 TWh – 148 TWh[40].
A breakdown of Scotland's energy demand by sector is shown in Figure 19. These are ranked in terms of likelihood to transition to hydrogen based on stakeholder feedback as discussed in Section 5. The darkest green represents areas most likely to transition to hydrogen, and lightest green most likely to be decarbonised by other means.
This gives an indication of the sectors that could be supported to grow early hydrogen demand, even if their overall contribution to energy demand is small. For example, a transition within rail and buses to hydrogen can be an early route to creating demand. However, by 2045, the total amount of hydrogen used in those sectors makes them a small overall contributor.
The carbon emissions from these sectors shown in Figure 20, provides a view of the relative carbon intensity of each sector. Heating makes up for over 50% of Scotland's energy demand, but only accounts for approximately 20% of carbon dioxide emissions. Transport accounts for approximately 25% of energy but generates over 35% of all carbon emissions. As such decarbonising relatively smaller proportions of our transport demands, will provide much more benefit in terms of reducing carbon emissions.
“Supply of hydrogen and demand must happen together and this can be challenging without coordination”
Stakeholder questionnaire opinion
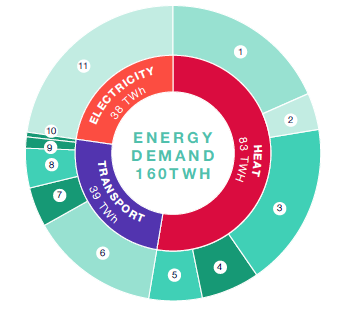
1. Commercial and industrial non-gas
2. Domestic non-gas
3. Domestic gas
4. Industrial gas
5. Commercial gas 6. Diesel and petrol cars
7. Heavy goods vehicles (HGV)
8. Diesel light goods vehicles (LGV) 9. Buses
10. Rail
11. Electricity

1. Heat
2. Electricty
3. Transport
4. Others
Hydrogen production potential
Scotland has the potential to produce significant quantities of both green and blue hydrogen. An ambitious view of Scotland in the next 20 years could see the country becoming an exporter of hydrogen to the rest of Europe. However, this is dependent on market conditions and ability to realise cost reductions.
Comparing blue and green hydrogen production
A blue hydrogen production plant rated at 1 GW will produce significantly more than a 1 GW green hydrogen production plant. Electrolysers quote their maximum energy capacity at the input of the electrolyer and SMR/ATR quote a value at the output of the SMR/ATR.
A 1 GW offshore windfarm, operating at a 58% capacity factor connected to a 1 GW electrolyser with an electrolyser conversion efficiency of 69% would produce 3.5 TWh of green hydrogen (92,000 tn) from the 5.1 TWh of input electrical energy.
A 1 GW blue hydrogen production facility operating with a capacity factor of 86% and a conversion efficiency of 80% would produce around 7.5 TWh (225,000 tn) of hydrogen in a year. This requires around 9.75 TWh of natural gas.
Appendix A2.1 provides more detail.
Green hydrogen production
There are two key factors that limit the potential for growth of green hydrogen production in Scotland: renewable energy generation capacity and green hydrogen plant/electrolyser build out potential. While the former is more directly influenced by UK and Scottish government policy, the latter is likely to be strongly influenced by what happens in a global market.
It is unlikely that renewable electricity resources will be the limiting factor in green hydrogen production, given the scale of potential resource and maturity of the market. It is more likely that the scale up and practical build out rates of green hydrogen production plant/electrolysers, will dictate the installed capacity of green hydrogen production achieved by 2045.
The most ambitious scenario in this assessment assumes that Scotland could credibly reach an installed capacity of 5 GW by 2032 and over 35 GW by 2045. This assumes strong global market support for hydrogen production which stimulates the supply chain.
Renewable energy potential
Scotland has some of the world's best renewable energy resources including 25% of Europe's offshore wind resource, extensive wave and tidal resources and over 60% of the UK's onshore wind capacity[43].
In a supportive planning and fiscal regime approximately 800 MW/year of renewable electricity was installed over the last decade to reach a total installed capacity of 11.9 GW, the vast majority of which is onshore wind[44]. The newer and fast growing offshore wind industry now has just under 1 GW of installed capacity[45].
The ultimate potential for renewable energy capacity in Scotland is difficult to estimate, as theoretical potential is limited by what is environmentally and commercially viable, as well as practical build out rates. However, the ultimate potential in Scotland is considered likely to be significantly larger than Scotland's and even the UK's indigenous demands[46].
It is likely that offshore wind will grow to take an increasingly larger proportion of new build capacity. The CCC have estimated that the UK may require up to 75 GW of offshore wind by 2050 to meet UK demands, reaching build out rates of up to 4 GW/annum[4]. In Scotland there is 8.4 GW of onshore wind in planning or construction, compared to 7.5 GW of leased offshore wind in the pipeline[47,48]. A further 10 GW of offshore wind is anticipated to be leased through Crown Estate Scotland's ScotWind leasing round. Longer term, wave and tidal have the potential to contribute to installed capacity and the Offshore Renewable Energy Catapult predict that in the UK as a whole 1 GW of tidal energy could be installed by 2030, and 1 GW of wave energy by 2040[49]. However, there is limited visibility in the pipeline of renewable projects beyond 2030.


Hydrogen may help unlock renewable energy resources that previously were not economically viable by offering a more diverse route to market and alternative revenue streams, by better use of existing infrastructure, and by separating production locations from demand locations.
For onshore wind, wave and tidal this could include development in areas of grid constraint, improving system flexibility and management of local supply and demand. For offshore wind, integration with O&G infrastructure could improve project economics. Hydrogen has the potential to play a critical role in supporting a Scottish offshore wind market as Scotland's challenging technical conditions and unfavourable grid charging regimes have resulted in Scottish projects being at a disadvantage to projects in the rest of the UK.
The assumed maximum build out rates of green hydrogen production of 5 GW by 2032 and 35 GW by 2045, are not likely to exceed what could be theoretically constructed in Scotland given the right market conditions.
The locations of Scotland's renewable resources are shown below. Scotland's existing offshore wind projects are largely clustered in the Firth of Forth and the Moray Firth off Scotland's east coast. However, ScotWind leasing rounds and the wave and tidal resources are more widely distributed round the Scottish coastline. Significant renewable potential is held in the islands and rural areas, including the Western Isles, Orkney and Shetland, that are grid constrained.
These resources could be used to produce electricity and/or hydrogen at point of generation or support centralised production of hydrogen onshore. Optimising the system economics and driving down the costs of green electricity production will be critical, as electricity cost constitutes a large percentage of the end cost of hydrogen.
Green hydrogen plant potential
Green hydrogen plant/electrolyser build out rates are likely to be the main constraint for scale up of green hydrogen production.
There are practical constraints in how quickly a market is likely to scale up, due to supply chain capacity constraints and investment market confidence. In order to secure investment in multi GW projects, the supply chain will need to ramp up capacity, and investors will need to be comfortable with the technology and market risks. These are likely to progress in parallel, if a market demand is established, then secured orders will allow cost reductions in technology to be achieved, investors will become more comfortable with risk and the projects can grow in scale.
However, this pathway is dependent on reducing the cost of renewable electricity in the Scottish market, which will strongly influence the production costs of hydrogen. It is also highly reliant on the global market stimulating demand for hydrogen production systems, as optimal cost reductions are unlikely to be achieved through supply of a Scottish market in isolation.
The rate of growth of the green hydrogen production market, is highly influenced by the strength and consistency of the market support. The UK offshore wind market grew from sub 100 MW projects around the turn of the century, to installing approximately 800 MW per year in cumulative capacity in just over a decade50. This was enabled by relatively stable market support regimes.
Currently the global electrolyser market is relatively small and the largest electrolyser systems that are being built are around 10 MW scale. Scotland has a modest pipeline of identified green hydrogen production projects. Figure 22 shows how the potential green hydrogen production capacity could be built up around Scotland.
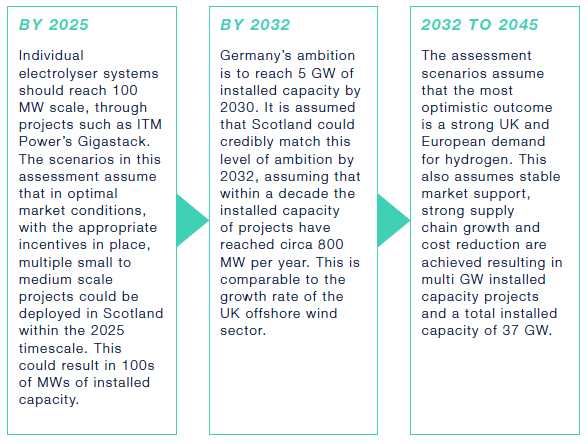
Blue hydrogen production
There are a number of factors that potentially limit the growth of blue hydrogen production in Scotland. Similar to green production, access to feedstock, in this case natural gas, and practical build out rates of blue hydrogen production plant are key. Moreover, matching supply to demand, investment periods, compatibility with carbon targets and competition with green hydrogen production have an impact.
While increasing blue energy production will create an increasing reliance on natural gas imports, this is not considered to be a constraint. It is considered more likely that the scale up of supply will be matched to meet indigenous demand, but not an export market. This will ultimately be curtailed when green hydrogen cost reductions mean the price of green drops below the price of blue (given that the latter is not zero carbon).
The most ambitious scenarios in this assessment assume that Scotland could credibly reach an installed capacity of 200 MW by 2025, 2 GW by 2032 and 5 GW by 2045.
Supply of natural gas potential
Natural gas is the feedstock of blue hydrogen production and so securing a supply is critical to investment in blue hydrogen production.
Scotland plays a key role in the supply of natural gas to the wider UK system, both from its indigenous production, but also as an importer from the wider North Sea gas network. The total flow of gas through St Fergus, both imports and indigenous, varies on an annual basis, and in 2019 was approximately 240 TWh, almost four times Scotland's current natural gas demand.
However, the UK Continental Shelf (UKCS) is an ageing basin and the total production of natural gas is in decline. Predicting the remaining economically viable reserves in the North Sea is challenging. It is possible that a hydrogen market, and more integration of offshore renewables with O&G assets, would increase the economic viability of reserves and slow the decline, thereby increasing overall production. The UK Government's 'Maximising Economic Recovery' strategy[52] seeks to slow the decline. However, Oil & Gas UK predict that by 2045, UK production will be approximately 30% of 2020 production53.
The majority of the UK's natural gas supply already does not come from indigenous supply. In 2018 the UK imported 54% of its total natural gas demand from global markets[54]. The proportion of UK gas imports will increase over time, if reliance on gas does not reduce as indigenous reserves declines.
The 'Further Ambition' scenario from CCC's Net Zero Technical report[3], assumes that up to 225 TWh of blue hydrogen could be required to achieve net-zero targets, but also assumes that it is achieved through increasing natural gas imports. It is therefore likely that blue hydrogen production at scale, will not be limited by availability of natural gas feedstock, but will result in an increasing reliance on imports.
Blue hydrogen plant potential
The Further Ambition scenario from CCC's Net Zero Technical report[3], assumes that up to 30 GW of methane reformation capacity will be constructed in the UK by 2050, assuming build out rates grow to 2-3 GW per year.
In Scotland scale up of blue hydrogen is likely to be demand driven, rather than supply driven. As blue hydrogen is not zero carbon and produces a lower purity hydrogen, compared to green production, it is unlikely that there will be an export market. This is supported by recent German and EU commitments to secure a green hydrogen network.
Blue hydrogen is therefore likely to be limited to use within the domestic gas network, or to meet local industrial demands. This is the logic of early blue hydrogen production projects like Acorn, which will see 200 MW of production installed in around 2025 to match 2% injection into the national gas transmission network[55]. Figure 23 shows how the potential blue hydrogen production capacity could be built up around Scotland.
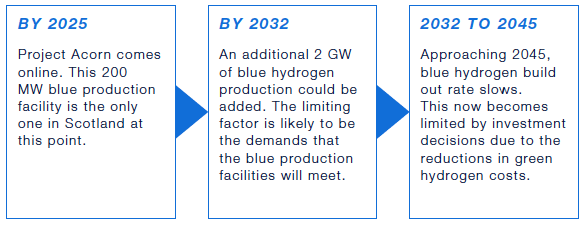
4.4 Innovation and academia
Scotland, the UK and the EU have complex structures of support for the development and innovation of energy technologies, from early stage fundamental research, right through to commercialisation.
A number of Scottish organisations are already taking a supporting role in the hydrogen economy, focusing on various stages of development. These include partnerships between industry, academia and public sector such as NECCUS[56], focused on supporting blue hydrogen through CCUS, and DeepWind[57], supporting green hydrogen through offshore renewables. However, there remains limited support for funding or partnerships focused wholly on hydrogen.
Scotland's universities have long played a role in supporting the national energy industry. Research and development (R&D) conducted in universities has a key role in developing innovative solutions and enabling technology development. Scotland's academic institutions are currently conducting some world leading academic work on hydrogen, such as HyStorPor[58]. This research is funded from a wide range of Scottish, UK and European funds.
The newly launched Hydrogen Accelerator will seek to draw on collaborations between universities, public sector and industry to support the early development of technologies and other hydrogen solutions in the transport sector[59]. Figure 24 shows Scotland's key academic skills and strengths in the hydrogen sector. More detail on this is available in Appendix A2.3.
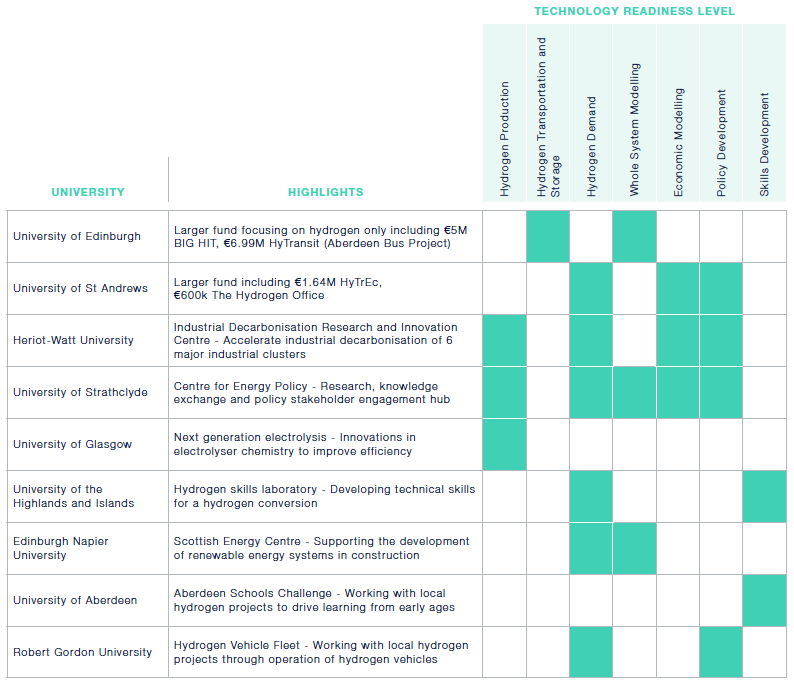
Figure 25 and Figure 26 provide a summary of some of the key funding opportunities and partnerships for hydrogen and where on the innovation spectrum these are targeted.
Despite the current focus, there is no overarching organisation that is co-ordinating the Scottish or UK research and innovation efforts focused on hydrogen. There are particular challenges in bringing together elements of funding that can support end to end projects that include production, distribution and demand. Targeting innovation funding on areas that align with Scotland's skills and supply chain can help capture more economic benefits.
Scotland could benefit from a strategic focus on where interventions in supporting innovation should be focused to best leverage benefits across the hydrogen value chain.
Engagement with industry suggested a desire to create an organisation which would co-ordinate innovation in a similar way to the role of the Catapults, Oil and Gas Technology Centre (OGTC), and H2FC Supergen.
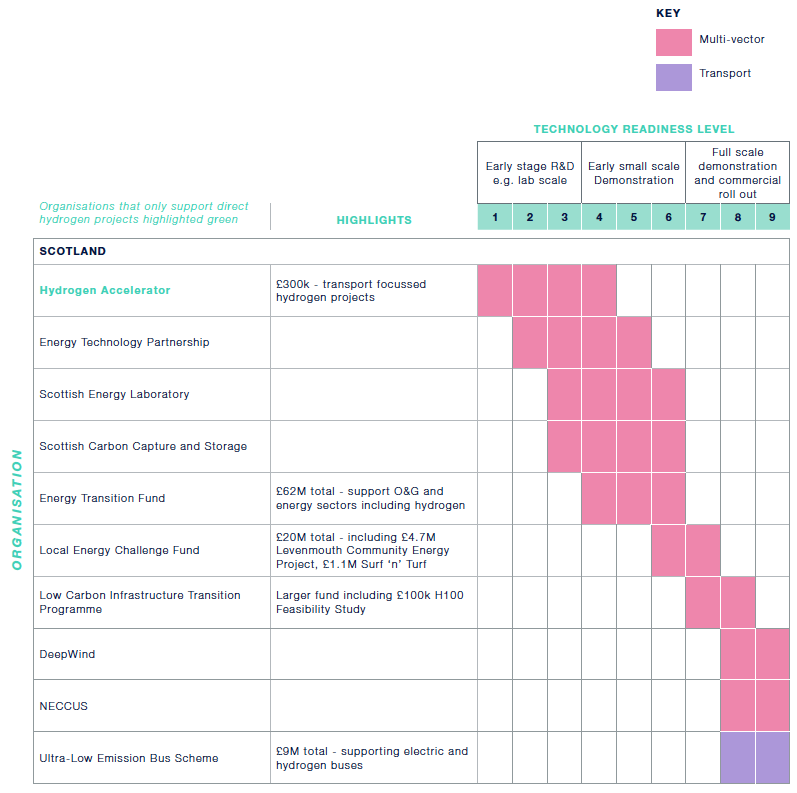


Contact
Email: onshoreoilandgas@gov.scot
There is a problem
Thanks for your feedback