Dairy Sector Climate Change Group: report
Report from the Dairy Sector Climate Change Group, one of the farmer-led groups established to develop advice and proposals for the Scottish Government. It focusses on how to cut emissions and tackle climate change, something that was re-emphasised in the updated Climate Change Plan.
6. A Balancing Act
Achieving net zero will require realigning the balance between the negative (GHG emissions and emissions intensity) and the positive (carbon storage and sequestration). We need to look at the activities across the whole farm as well as collaboration and interactions on a community and regional basis to realign the balance.
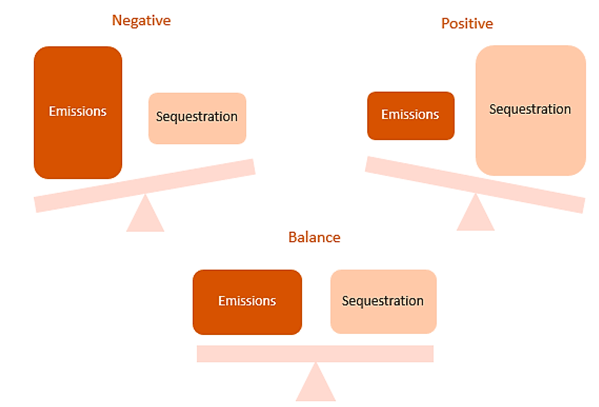
6.1 Minimising the Negative - Mitigation and Abatement
Figure 7 below illustrates how the emissions from dairy break down by source. Enteric fermentation (the process in which livestock produce methane via digestion) is the largest source contributing to 45% of dairy emissions. The next largest source is manure management.
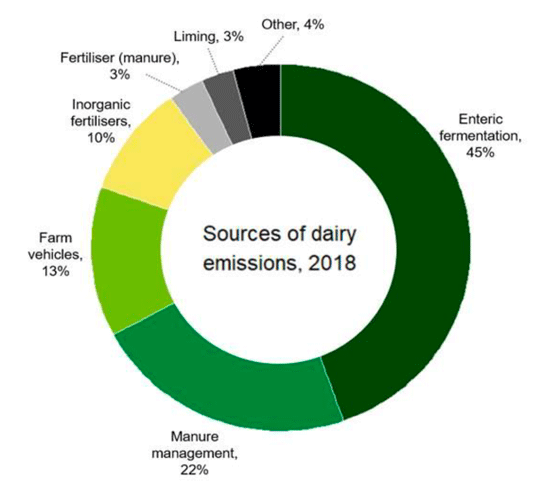
According to the CCPu, the Scottish Government anticipates that by 2032 the agriculture sector will have adopted and be competently using all available low emission technologies throughout the whole sector. Scottish Dairy's "Rising to the Top 2030"[24] supports this ambition and sets staged targets along the way, (Figure 8). This will require full buy-in from the dairy sector as well as alignment of policy, funding and other forms of advice and support.
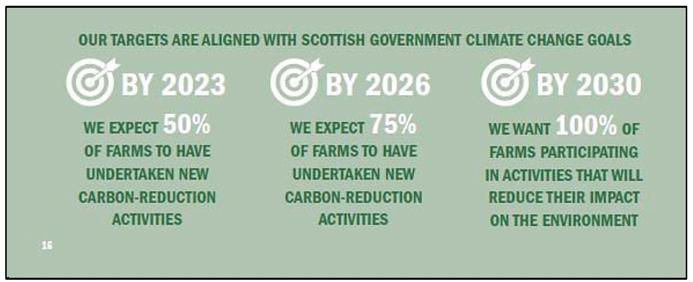
Graphic text below:
Our targets are aligned with Scottish Government climate change goals
By 2023 we expect 50% of farms to have undertaken new carbon-reduction activities
By 2026 we expect 75% of farms to have undertaken new carbon-reduction activities
By 2030 we want 100% of farms participating in activities that will reduce their impact on the environment
There are numerous potential ways of reducing emissions in the agriculture and land use sector. A review in 2015, identified 181 separate mitigation measures[25]. Of these, 71 measures were reviewed by a group of experts considering the likely abatement potential, practical feasibility, and the risk of negative co-effects and 24 measures were selected for further analysis, along with 7 additional measures.[26].
Most recently, the Climate Change Committee in their 6th Carbon Budget[27], published in December 2020, and with reference to ongoing research by Defra (Delivering Clean Growth through Sustainable Intensification), this has been further focussed into 18 measures, with 15 of these relevant to dairy farming. These measures are summarised in Figure 9 below (shown in grey). Additional measures have been included from work commissioned by AHDB[28] and the Climate Exchange Report into mitigation technologies and practices in Scotland[29] (both shown in white). The measures in Figure 9 outlined in green have been identified as not included in the 'smart inventory' or more Scottish data is required to improve accuracy.[30]
Reports form the other farmer led sector groups will also summarise various mitigation measures with varying eight attributed to them depending on the sector. There is a significant degree of overlap therefore between the sectors when it comes to mitigation. We have tried to focus on those measures most relevant to the dairy sector but knowledge can be drawn from the other farmer led reports too.
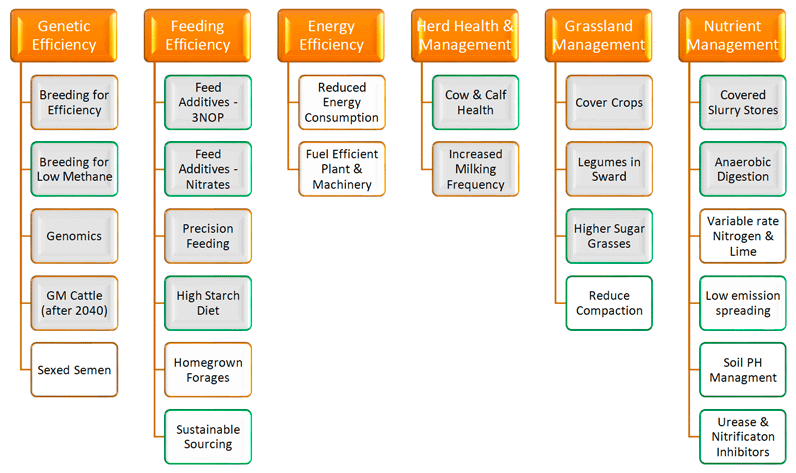
Graphic text below:
Genetic Efficiency
- Breeding for Efficiency
- Breeding for Low Methane
- Genomics
- GM Cattle (after 2040)
- Sexed Semen
Feeding Efficiency
- Feed Additives 3NOP
- Feed Additives - Nitrates
- Precision Feeding
- High Starch Diet
- Homegrown Forages
- Sustainable Sourcing
Energy Efficiency
- Reduced Energy Consumption
- Fuel Efficient Plant & Machinery
Herd Health & Management
- Cow & Calf Health
- Increased Milking Frequency
Grassland Management
- Cover Crops
- Legumes in Sward
- Higher Sugar Grasses
- Reduce Compaction
Nutrient Management
- Covered Slurry Stores
- Anaerobic Digestion
- Variable rate Nitrogen & Lime
- Low emission spreading
- Soil PH Managment
- Urease & Nitrificaton Inhibitors
6.1.1 Genetic Efficiency
Genetic improvement of cattle produces permanent and cumulative changes in performance, by improving productivity and efficiency, reducing wastage in the farming system and directly selecting on emissions. Selecting on traits that improve the efficiency of the system (e.g., feed conversion efficiency, longevity) will also have a favourable effect on the overall emissions, assuming no increase in production.
There has been widespread uptake of genetic improvement in the Scottish dairy herd, with the use of artificial insemination, increasing use of sexed semen and more recently genomic testing. This improvement in genetic potential has led to a decline in the national herd and an increase in milk output. Direct selection for reduced GHG emissions continues to rely on selection of traits that have a correlated effect on emissions, but increased research into direct methane emissions will help improve this.
The increasing use of sexed semen is improving efficiency by reducing wastage (male dairy calves), increasing the proportion of pure dairy calves that are female and increasing the number of beef cross calves for rearing as beef animals. Increasing the number of beef cross calves means that fewer suckler cows are required to produce the same total beef output, thereby reducing the total emissions and the emissions per kg of beef produced.
Genomic testing of youngstock gives greater accuracy in selecting youngstock from which to breed replacement heifers, allowing evaluation of the mixture of genes that have been passed down from both parents. Gene editing for production traits, health/resistance traits and potentially GHG emissions would speed up the traditional gene selection, and has been identified by the Climate Change Committee as a mitigation measure post 2040.
Recommendations:
- Consideration of support for genomic testing as part of a breeding programme
- Prioritised research into breeding selection for GHG emissions including an examination of any barriers to use breeding indices e.g. not matching farming goals.
Refer to Annex 4 – Recommendations and Support Required
6.1.2 Feeding Efficiency
Enteric emissions of methane are the largest source of GHG emissions from dairy herds, contributing to 45% of their emissions. Adapting feeding strategies can help reduce emissions through:
- Precision Feeding – In-parlour/robot feeding and/or out-of-parlour feeding, targeted at individual cow dietary requirements (e.g., stage of lactation). Solutions likely to be system and farm specific.
- High Starch Diet – A high starch diet increases the digestible energy content of the diet and is achieved by feeding more maize silage and reducing grass silage. However, maize cultivation area in Scotland is limited so more work is required to explore alternatives such as whole crop or other starch sources suitable to Scotland.
- Feed Additives - 3NOP is a chemical that reduces the excretion of enteric methane by ruminants when added to their feed ration or introduced via a bolus, however, is not yet approved for use. Nitrates can also be added to TMR (total mixed rations) to reduce emissions. More recent research into feeding the seaweed Asparagopsis taxiformis has had very positive methane reduction results, although the environmental effects of sourcing the seaweed needs to be looked at too[31].
- Improving Home-Grown Feed Quality – Improving the digestibility and quality of home-grown forages will increase feed conversion efficiency and in turn increase emissions efficiency and increase profitability.
- Sustainable Sourcing of Feeds – Sustainability of purchased feed will improve the life cycle analysis of dairy products and dairy beef. Sourcing locally will reduce transportation, utilisation of co- and bi-products will reduce waste and sustainable sourcing of imported feed will reduce environmental costs. However, local sourcing will only improve GHG emissions if the feed is produced as GHG efficiently as the non-local alternatives, given that transportation emissions are small part of the emissions.
- With the urgency of delivery on emissions abatement and the evolving science, contingency should be made for some new measures to have negative productivity outcomes e.g. feed additives, methane inhibitors, rumen microbiome adjustments. This would reduce emissions efficiency but may reduce absolute emissions and compensation for income foregone in these circumstances should be allowed for.
Recommendations:
- Capital support for feeding efficiency measures
- Robust research into feed additives for reduced methane emissions in Scottish herds
- Prioritise research into alternatives to maize such as whole crop or other varieties suitable to Scotland.
Refer to Annex 4 – Recommendations and Support Required
6.1.3 Energy Efficiency
Dairy unit power requirements are generally high. Since electricity production emits carbon dioxide as opposed to more potent climate change gases such as methane and nitrous oxide, the impact on the dairy unit carbon footprint is less. However, there does remain some opportunity to reduce emissions from the dairy unit and potentially significant cost savings, such as more effective milk cooling, heat recovery units, matching equipment size to demand, checking insulation and thermostat settings, variable speed vacuum/milk pumps, LED lighting etc.
There are also opportunities to reduce fuel consumption on farm with fuel efficient machinery, efficient use of machinery (e.g., reduced idling time, optimising power bands) and in the future, use of alternative fuel sources e.g., electric, biofuel, hydrogen etc. The role of contractors in delivering the fuel efficiency outcomes also needs to be considered. Smart recording apps for machinery and contractors will help measure outcomes.
Recommendations:
- Capital support for energy efficiency investments
- Removal of barriers to renewable energy investment on farm
Refer to Annex 4 – Recommendations and Support Required
6.1.4 Herd Health and Management
Improving herd health is a very broad measure, encompassing a variety of livestock management, disease prevention and treatment options. Endemic cattle diseases have a negative effect on dairy cattle production and productivity, and consequential impacts on GHG emissions. This typically stems from: increased mortality, depressed milk production, increased waste from discarded treatment milk and reduced reproductive performance. IBR (Infectious Bovine Rhinotracheitis), Salmonellosis and Johne's disease all present challenges for the dairy sector.
Mastitis is globally the most economically significant disease of dairy cattle, and if controlled has the potential to reduce GHG intensity in UK herds by 6% on average, and 12% for the worst affected of herds. Similarly, improved reproductive performance through managing infertility could reduce GHG intensity in UK herds by 7% on average and 16% on the worst affected herds.[32] Successful treatment of lameness has also been shown to reduce emissions intensity by 1-8%, depending on the prevalence of disease[33]. Aside from the obvious welfare gains, improved cow health also increases its longevity, reducing replacement rates and improving its climate efficiency.
Health of youngstock is also important to ensure heifers calve down at 24 months and beef cross calves finish as early as possible, thereby reducing their emissions intensity.
There are very significant challenges in accounting for animal health improvement measures within current inventory reporting methods. However, authenticated key performance indicators could be collated to allow the effects to be included[34].
Improving herd health improves productivity and profitability. Similarly, increasing the milking frequency from twice to three times a day can reduce nitrous oxide emissions. More milking increases the nitrogen utilisation of the cow, which leads to a fall in nitrogen excretion[35]. Milk yields would increase, although components could reduce partially offsetting the gains when expressed as kgCO2e/litre energy correct milk (ECM).
Recommendations:
- Capital support for health and fertility improvement measures.
- Capital support for robotic milking systems and parlour improvements to allow 3x milking.
- Development of key performance indicator matrix and collation to inform inventory and measure improvements.
Refer to Annex 4 – Recommendations and Support Required
6.1.5 Grassland Management
Improved grassland management to increase grass yields will reduce emissions intensity and potentially reduce the land requirement for grass, providing opportunity for sequestration and biodiversity land use. In addition, specific mitigation measures have been identified for grassland:
- Cover crops – These are crops sown after harvest of cereals, oil seed rape and other arable crops harvested in late summer. Catch/cover crops may be grown to reduce the risk of nitrate leaching over winter, reduce the risk of soil erosion, improve soil structure, increase carbon sequestration and provide a source of N to the subsequent spring-sown crop.
- Legumes - N fixing crops (legumes) form symbiotic relationships with bacteria in the soil that allows them to fix atmospheric N and use this in place of N provided by synthetic fertilisers. In the legume-grass mixtures the leguminous crops (e.g. white clover) can provide a substantial part of the grass's N requirements, reducing the need for N fertilisation. This measure is about increasing the legume-grass mix areas on grasslands and increasing the proportion of legumes in the mixture. The benefits of multi-species swards should also be explored, not only for potential carbon benefits but also biodiversity.
- High Sugar Grasses - Perennial ryegrass diploids with elevated concentrations of water-soluble carbohydrate (WSC), commonly termed 'high-sugar grasses' (HSGs), have been promoted as a tool for increasing the efficiency of the use of protein (N) in the rumen and thus offering scope for increasing milk production and animal growth rates, while reducing N losses (in the form of urine) to the environment.[36]
- Soil Compaction – Compaction of soils has been reported to increase N2O emissions and strongly reduce the soil's ability to sequester carbon (see Carbon Sequestration). Prevention of soil compaction requires better planning of field operations to avoid traffic on wet soil, avoiding or strongly reducing tillage of wet soil and reducing stocking density.
Recommendations:
- Research and demonstration of best grassland management practice within different dairying systems in Scotland, with capital assistance for equipment needed.
Refer to Annex 4 – Recommendations and Support Required
6.1.6 Nutrient Management
Organic manures applied to agricultural land are valuable sources of organic matter and plant nutrients. Careful storage, sufficient capacity and precise application to land allows their nutrient value to be used for the benefit of crops and soils, and significant reduction in the use of inorganic fertilisers.
- Covered Slurry Stores – Animal excreta stored in liquid systems is a source of substantial ammonia and methane emissions. Several factors affect the rate of ammonia, methane and nitrous oxide emissions, including the airflow over the manure; by covering the stores these emissions can be reduced. The presence of a slurry cover increases the ammonia concentration in the slurry, and hence its nitrogen and fertiliser value.
- Anaerobic Digestion - Anaerobic digestion (AD) of slurries has a GHG reduction potential outweighing that from improved storage of slurries and manures. Methane emissions from the storage of slurries and manures are reduced and the methane generated from livestock manures during AD can be used to produce heat and power to replace fossil fuel use. In addition, there is the potential to increase nitrogen use efficiency and reduce the required quantity of manufactured fertiliser if the digestate is subsequently spread to the land. However, significant start-up and running costs are barriers to uptake.[37]
- Variable Rate Applications - The use of techniques such as variable rate fertiliser and lime spreading and GPS soil testing can lower the amount of excess nitrogen applied to fields. This in turn can reduce the GHG emissions as well as reducing risk of nitrates entering watercourses through run off and limit the impacts on air quality through reduced ammonia emissions.[38] Precision lime application takes account of often large gradients in pH within fields, applying lime with variable rate applicators on a spatial basis according to pH balancing requirement.
- Low Emissions Spreading – Slurry application using techniques such as bandspreading and injection, reduce emissions compared to splash plate spreading. Ammonia emissions are mitigated, although direct emissions of nitrous oxide can increase.
- Soil pH Management – Soil surveys in Scotland show that many areas have soils that are too acidic, which compromises crop growth, reducing yield and increases the proportion of nitrogen fertiliser emitted as nitrous oxide. Soil pH requires regular soil testing and lime applications where necessary.
- Urease and Nitrificaton Inhibitors – Nitrous oxide emissions from nitrifying bacteria can be inhibited by certain chemical compounds, which increased the nitrogen availability in soils and reduces nitrous oxide emissions. Similarly, ammonia emissions can be generated by bacterial action on urea-based fertilisers. Inhibitors applied with fertilisers or mixed into slurry prior to application can reduce emissions.[39]
Recommendations:
- Capital support for increased slurry storage, slurry store covers, low emission spreading equipment, variable rate application equipment, flexible tyres.
- Support for soil sampling to enable field mapping and soil carbon measurement.
- Capital support for removal of barriers to anaerobic digestion for slurry and establishment costs.
Refer to Annex 4 – Recommendations and Support Required
6.2 Maximising the Positive - Carbon Sequestration
Mitigation alone will not achieve net zero in dairy farming. Gross emissions from cattle cannot be reduced to zero due to the natural biological processes such as enteric fermentation. However, carbon sequestration by the natural landscape and other approaches to remove GHG from the atmosphere can contribute to balancing the emissions[40]. This sequestration needs to be included in carbon auditing, recorded in the inventory and credited to the agriculture industry. However, it is widely acknowledged that more research and agreement on measurement is needed.
Recommendations:
- Prioritisation of research into methods of including measurement of carbon sequestration in carbon auditing tools, which could also inform inventory reporting.
Refer to Annex 4 – Recommendations and Support Required
6.2.1 Soil Carbon
Soils hold three times the amount of carbon currently in the atmosphere or almost four times the amount held in living matter. Because soils have such a large storage capacity, enhancing soil storage by even a few percentage points makes a big difference[41]. There are knowledge gaps and challenges that hinder the upscaling and widespread deployment of soil carbon management, as acknowledged by the IPPC, and addressing these issues must be an urgent priority[42].
Opportunities exist to use agricultural management to increase carbon storage in agricultural soils[43], for example through conservation management practices such as reduced cultivations and compactions. However, there are uncertainties in the amount of carbon that can be sequestered by restoration of organic soils, rotational grass, the future carbon sequestration potentials of long-term grasslands and arable soils[44], and further research is needed.
There is some evidence that suggests there have been no significant changes in the storage of carbon taking place in arable or grassland soils in 40 years. However, soil carbon can accumulate for over three decades with no evidence within permanent pastures, highlighting the complexity of the issue and the urgent need for greater understanding[45].
Nevertheless, it must be acknowledged that Scottish soils on many dairy farms are relatively rich in carbon, so the opportunities for further sequestration are more limited, although protection is vital. Opportunities to transfer organic carbon produced on dairy farms to soils with depleted levels, for example in more arable areas, need to be explored (see Figure 10).

Graphic text below:
Arable Cropping
- Approximately 40 45 T/Ha
Improved Grassland
- Approximately 60 65 T/Ha
Unimproved Grassland
- Approximately 80 90 T/Ha
Peatland
- Approximately 260 T/Ha
Mixed Woodland
- Approximately 65 T/ha (+ c.55 T/ha above ground)
Figure 10 also demonstrates that restoration of peat soils has the opportunity to sequester large amounts of carbon, and as such their protection and restoration is also vital. This measure will be restricted to those dairy farms with peatland soils.
Aside from the uncertainties, it is widely accepted that enhancing soil health through improved physical structure and microbial activity, will improve productivity and reduce inorganic inputs, as well as improved drought resistance and water retention. Research has shown that animal manures are the best approach to return carbon and improve soil health[46].
Recommendations:
- Demonstration of best practice in soil carbon management and soil health.
- Funding for restoration and protection of peat soils (where applicable).
- Prioritisation of research into soil sequestration measurement and improvement techniques on farm.
Refer to Annex 4 – Recommendations and Support Required
6.2.2 Afforestation
Enhanced carbon sequestration by trees on agricultural land can be achieved by afforestation, woodland management, agroforestry and hedgerow planting. Additional benefits of woodland on agricultural land can be the provision of shade and shelter, the reduction of ammonia emissions, enhancement of biodiversity, improved water management and potential additional income from fuel and timber production[47].
There is a strong evidence base for the sequestration potential from planting trees. However, as demonstrated in Figure 10, it is important that trees are planted in the right places to ensure carbon is not lost (e.g., not on peatland or unimproved permanent grassland). Tree planting on more productive arable and improved grassland delivers greater carbon benefits but is in competition with the agricultural productivity of the land and has long-term implications. Hedgerow planting and agroforestry can offer mutually beneficial outcomes.
Recommendations:
- Greater incentivisation of smaller scale tree and hedgerow/corridor planting, with the sequestration captured in the carbon audits and inventory for agriculture.
- Demonstration and practical advice for planting, for both biodiversity and carbon sequestration benefits.
Refer to Annex 4 – Recommendations and Support Required
6.3 Biochar and New Carbon Capture Technologies
Biochar is produced by treating organic matter with heat in low or zero oxygen environments (pyrolysis or gasification) to create a charcoal like product which can stabilise organic matter when added to soil. However, there are concerns of its use in UK soils and climate, and the feasibility of incorporating biochar into soils to the extent that it would have an impact on climate change, e.g. accumulation of heavy metals and other compounds[48].
Innovations in carbon capture technology and storage offer huge potential[49], for example, Direct Air Capture and Carbon Storage (DACCS) and Bioenergy with Carbon Storage (BECCS). They offer strong potential for carbon capture but at high relative cost[50], with further research needed before they offer viable solutions.
Recommendations:
- Research into the viability of the use Biochar in Scotland for climate change mitigation.
- Participation in international efforts to explore new and develop existing carbon capture technologies.
Refer to Annex 4 – Recommendations and Support Required
Contact
There is a problem
Thanks for your feedback